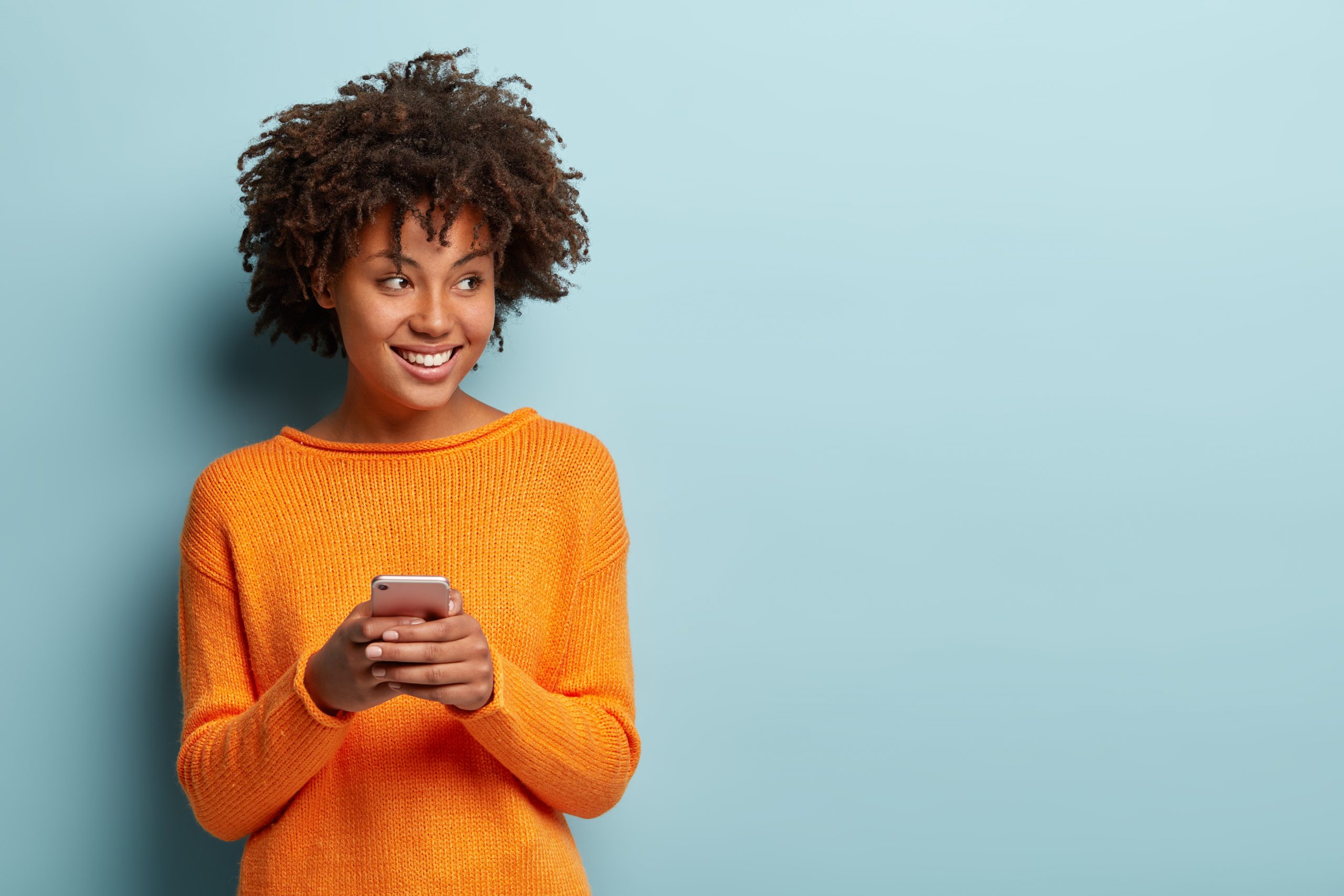
From watches to toothbrushes, mobile phones to cars, batteries are a power source for many of our everyday belongings. And while their beginnings can be traced back to the 19th century, their innovation has transformed industries, technology use and society at large today.
Energy storage systems such as pumped-hydropower have long played an important role in balancing electricity systems, but as the UK and countries around the world seek to decarbonise industries and make greater use of intermittent, renewable sources, there is a need for greater levels of storage.
While pumped-hydro storage requires the right kind of terrain, batteries can theoretically be built wherever there is the space and investment. But what actually is a battery, and how does it work?
Turning chemicals to electrical flow
Batteries are comprised of one or more cells which store chemical energy, and are able to convert that energy into electricity. In most batteries, there are three main components: an anode, cathode and electrolyte.
The anode and cathode are terminals for the flow of energy and are typically made of metal. The electrolyte is a chemical medium that sits between the terminals allowing an electrical charge to pass through. This is often a liquid, but increasingly research points to the potential to use solids and create what are known as solid-state batteries.
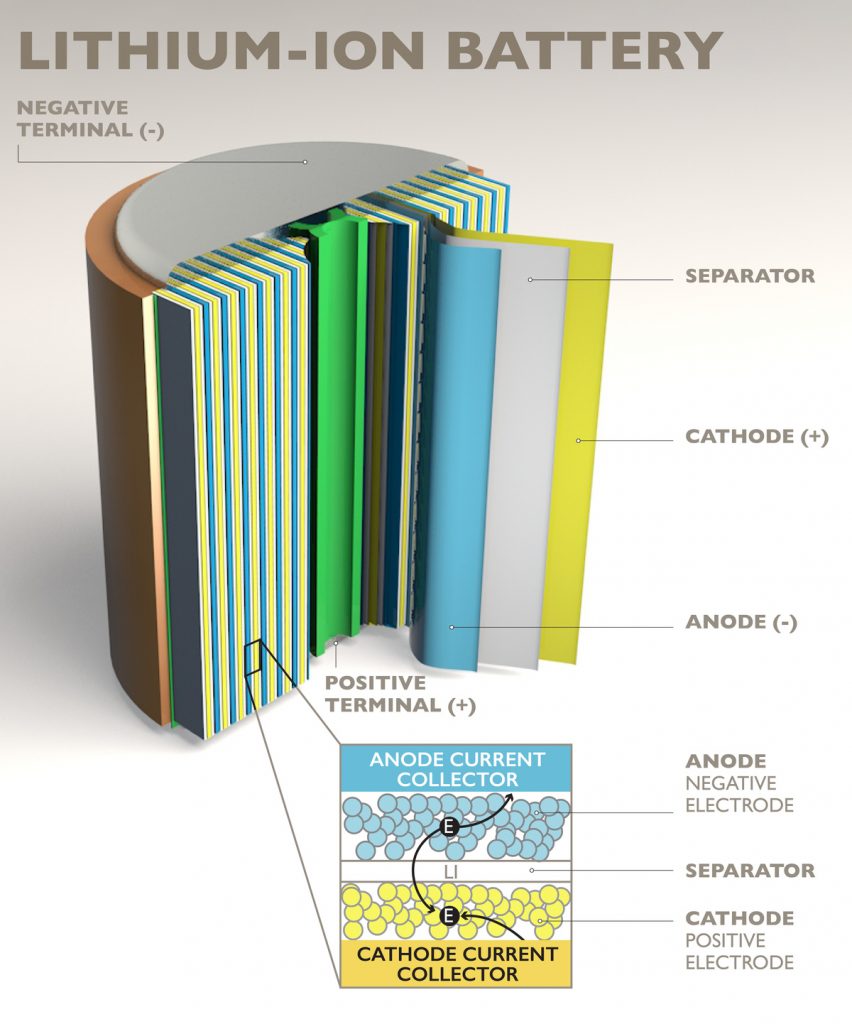
How a lithium ion battery works
It’s only when a battery is connected to a device that it completes a circuit and chemical reactions take place that allow the flow of electrical energy from the battery to the device. But how much electrical energy a battery can dispense has always been a hurdle to using them as a power source, making rechargeable batteries an important breakthrough.
The same reaction backwards
A key element in battery development was the exploration of rechargeable cells. These have long provided mobility and reliability in small scale outputs, but are now being looked to as a source of large-scale energy storage.
Invented by physician Gaston Planté in 1859, rechargeable batteries are possible because the chemical reactions that take place are reversible. Once the initial stored charge has been depleted via chemical reaction, these reactions occur again, but this time backwards, to store a new charge.
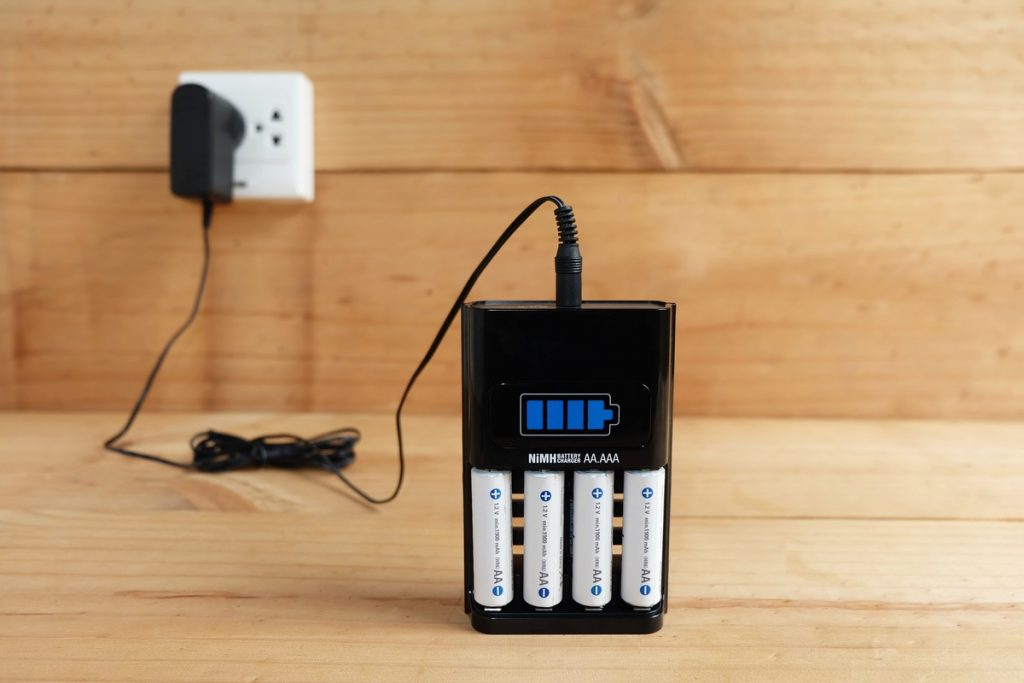
Battery charger with AA rechargeable batteries
Using a lead-acid system, Planté’s composition was similar to that found in rechargeable batteries used in cars and motorbikes today, although the characteristics of these cells, such as their heavy weight, meant they were not convenient for many other uses.
As a result, a journey of continuous research and optimisation to decrease the size and weight of rechargeable batteries began. This includes investigation into the alternative chemical compositions found in batteries today – nickel-metal hydride and lithium-ion to name two.
Recharging in a low-carbon energy system
Just as we have seen the size and capacity of batteries bettered throughout history, the application and optimisation of modern-day lithium-ion cells looks to continue too, powering the world’s move towards a low carbon, renewable energy future.
From electric vehicle batteries with a million mile lifespan to a 200 megawatt battery farm in South Africa, lithium-ion allows reasonably large-scale energy storage. It can also play a key role in power grid stabilisation over short durations of time such as a few hours.
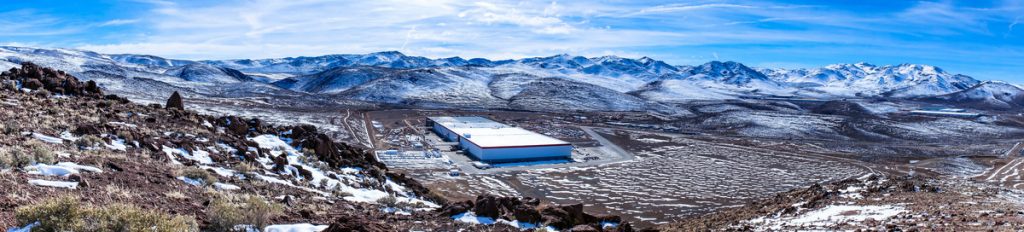
Tesla gigafactory
For the UK to run on 100% renewable electricity sources, batteries would be imperative in complimenting other flexible renewables, such as biomass and hydropower. As a support technology, batteries can help ensure a continuous supply of electricity to homes and cities, even when cloud cover and low wind prevents other sources generating.
Conversely, charging and recharging batteries can also be used to absorb and store electricity when there is more sun and wind generation than needed, avoiding surges in electric current or wasted generation.
Changing charging
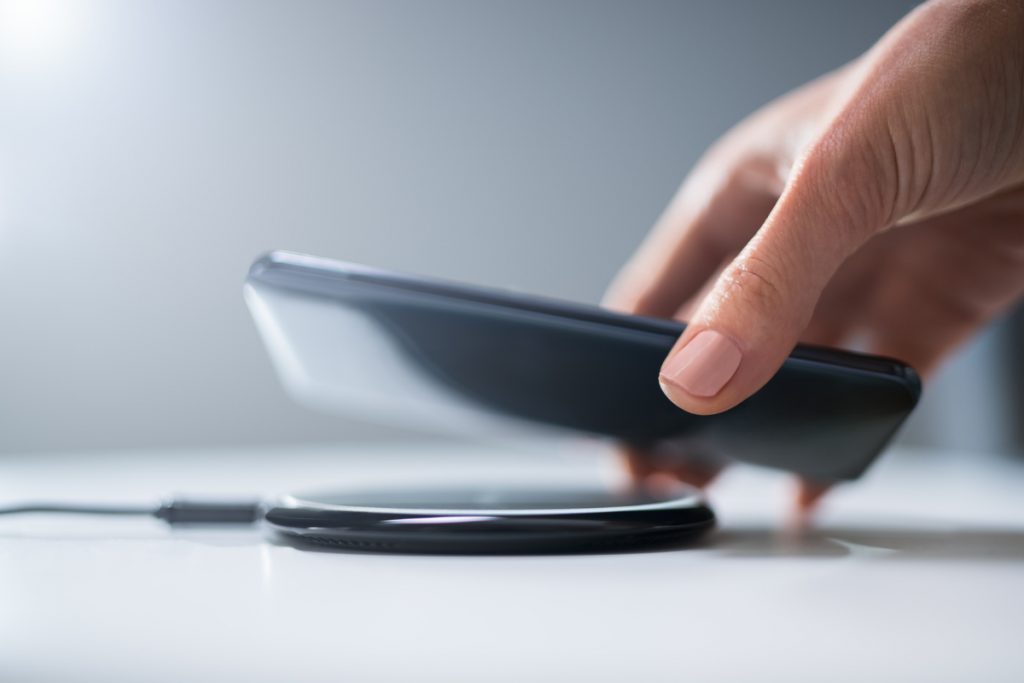
Woman charging smartphone using wireless charging pad
Alongside the advancements of battery capacity and composition, the way we use them to charge is also changing. Just as Bluetooth and Wi-Fi avoid the tethering required of wired connections, wireless charging can increase mobility and remove physical limitations.
Small-scale wireless charging is in use today. Many mobile phones, toothbrushes, smartwatches and earbuds now have wireless charging pads. These use near-field charging, meaning the device must be in close proximity to the charger to receive power.
However, efficient far-field charging is in development, with companies like Energous and Ossia developing over-the-air charging solutions for wearable tech, medical products, smart homes, and industrial equipment. This would mean devices could be powered and charged from many metres away.
The implications of this are vast, for example your devices could be charged just by entering your home or office. There could be less need for invasive surgery to change the batteries for pacemakers, neurotransmitters and other implanted medical equipment.
This type of technology could also provide passive charging for electric vehicles. The UK Department for Transport has announced a trial in Nottingham, where charging plates will be placed on parts of the town’s roads allowing electric taxis to charge while waiting briefly to pick up passengers. As charging technology and speed continues to increase, this might mean vehicles could charge wirelessly not only while parked, but when stopped at traffic lights.
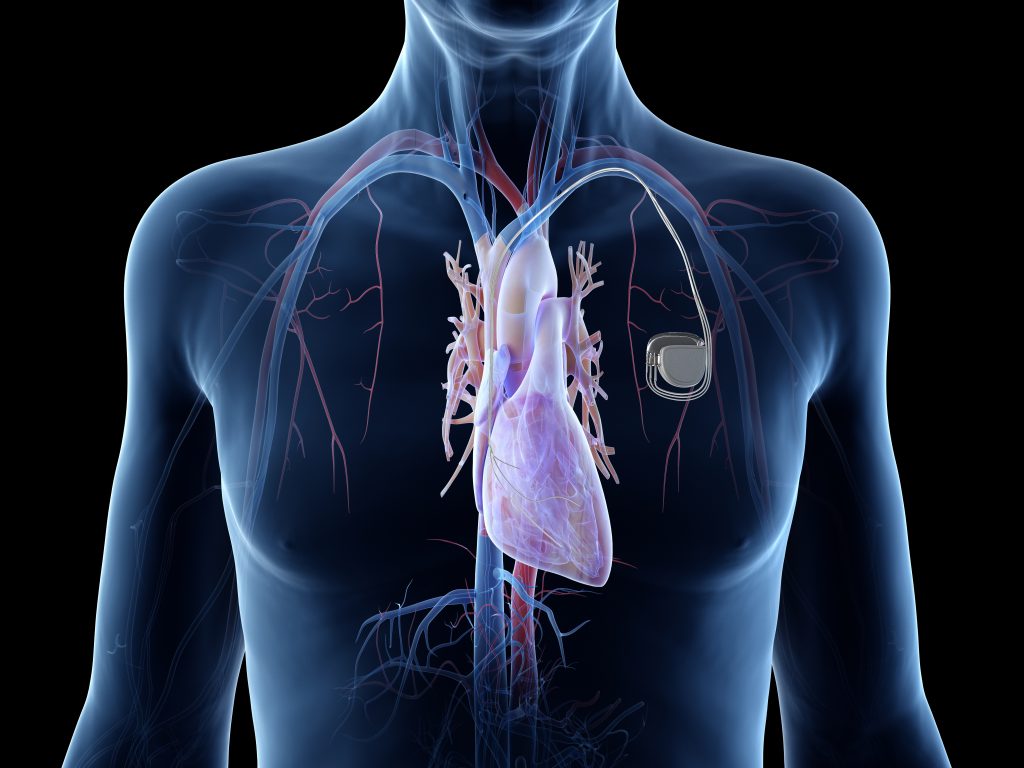
3d rendered illustration of an elderly man with a pace maker
As the world shifts away from fossil fuels to renewable sources, batteries, with continued improvement in performance and capacity, will be crucial in supporting our connected lives, transport systems and electrical grids.