It was no surprise when President Donald Trump echoed his election campaign stance and announced his intention to renegotiate – or failing that withdraw the US – from the Paris Agreement on Climate Change. It raised the question, would other countries back away from their own climate change targets?
In fact, many reaffirmed their commitment to the pact and continue their progress towards becoming low carbon economies. For those in the European Union, this means meeting the 2030 climate and energy framework, which sets three key targets for member states: cut greenhouse gas (GHG) emissions by at least 40% from 1990 levels, produce at least 27% of their energy through renewable sources, and improve energy efficiency by at least 27%.
Many countries across Europe, however, have set climate objectives that go beyond these. Whether they can meet those goals is another matter.
Portugal
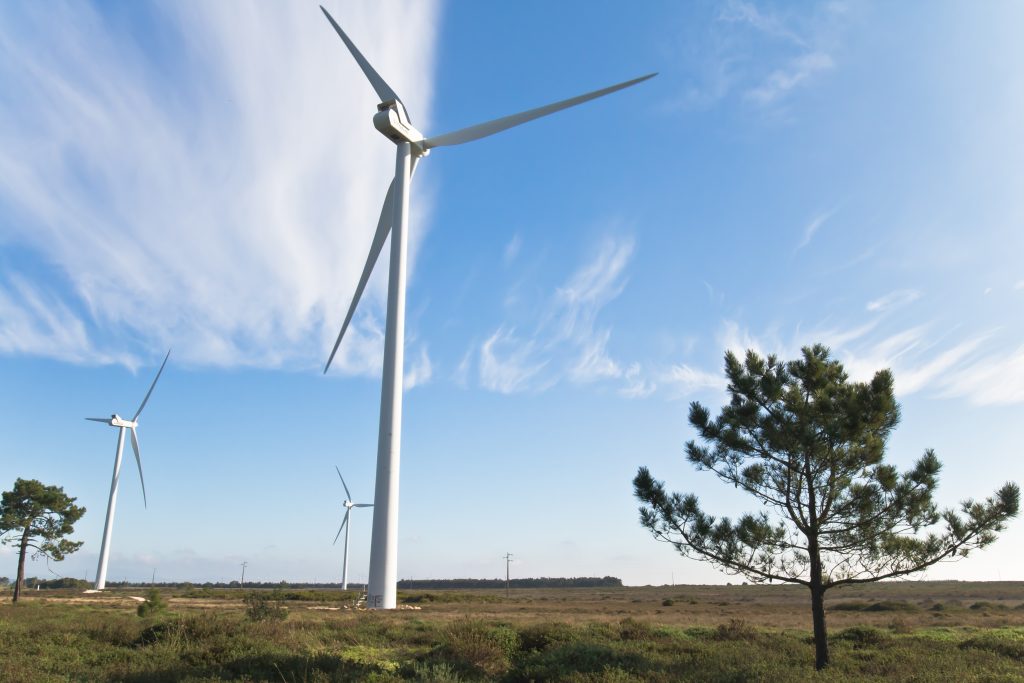
What are its climate targets?
The Portuguese government has pressed the EU to go further than its 2030 targets and is aiming for 40% of total energy consumption to come from renewables by 2030. This target is part of its Green Growth Commitment 2030, which also sets out to create more green – or low carbon economy – jobs and improve overall energy efficiency across the country.
How is it achieving this?
Portugal has rapidly increased its renewable energy production by investing in wind (mainly onshore) and hydro power, although it is rapidly developing its solar capabilities. It is also looking at small scale renewable energy generation through wave, thermal and biomass power.
Portugal has two operational coal plants that together are responsible for 16% of the country’s carbon emissions. However, the government is seeking to phase these out prior to 2025.
How is it doing so far?
The growth in renewable energy within the power industry specifically has been a big success story for Portugal. In 2005, renewables accounted for only 16% of total electricity production – by 2015 they produced an average of 52%.
The country made headlines in May 2016 for running on 100% renewable electricity for four days in a row. Unsurprisingly, this means the government is confident of achieving a target of 31% renewables in gross final energy consumption by 2020, which would mean 57.4% renewable electricity generation.
Germany
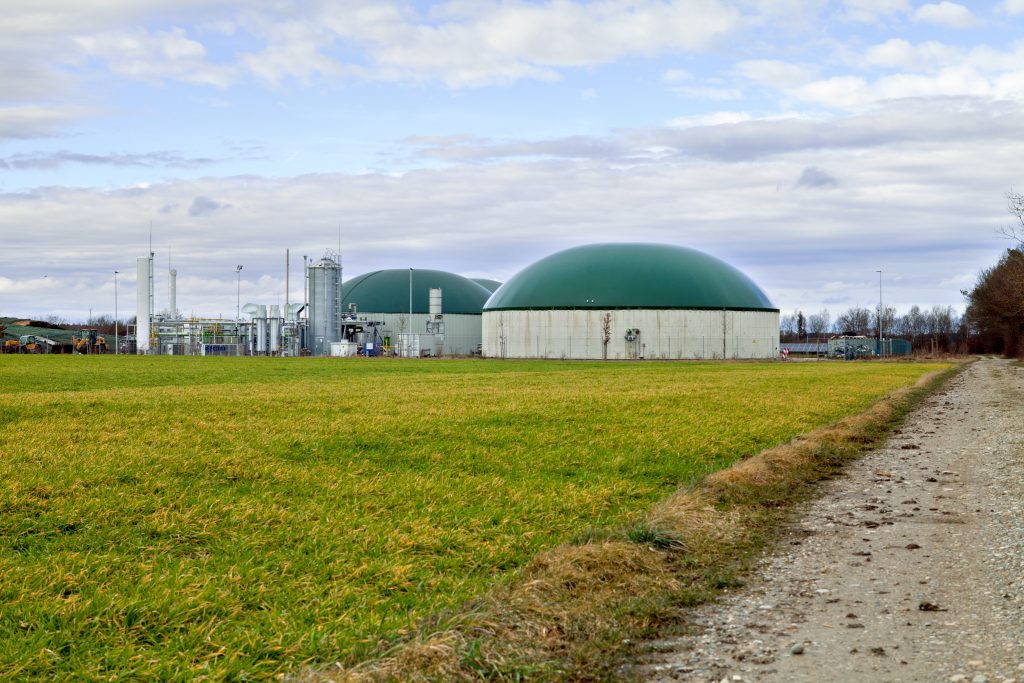
What are its climate targets?
Germany set its current climate targets as far back as 2007. It subsequently agreed to the Paris Agreement and the EU’s 2014 climate and energy framework.
Added to this, the country has its own ambitious aims for 2050: cut GHG emissions by up to 95% compared to 1990 levels (with an interim target of 40% by 2020), increase the share of renewables in gross final energy consumption to 60%, and increase all electricity generated from renewables to 80%.
How does it plan to achieve this?
Germany’s Climate Action Programme 2020 and Climate Action Plan 2050, set out its plans for reducing GHG emissions. Much of this is based around the Energiewende (energy transition), a strategy that will see the country phase-out nuclear power and decarbonise the economy through renewable energy initiatives.
According to these plans, Germany’s energy supply must be almost completely decarbonised by 2050, with coal power slowly phased out and replaced with renewables, especially wind power. The utilisation of biomass will be limited and sourced mostly from waste. It also stresses the role of the European Union Emission Trading System to meet targets.
How is it doing so far?
Between 1990 and 2015, emissions reduced by 27%. In 2015, the share of renewable sources in German domestic power consumption amounted to 31.6%.
However, German energy-related CO₂ emissions rose almost 1% in 2016, despite a fall in coal use and the ongoing expansion of renewable energy sources. This rise is due in part to an overall increase in energy consumption and an increase in natural gas use and diesel for electricity, heat and transport.
Projections from the environment ministry in September 2016 indicated that Germany will likely miss its 2020 climate target.
UK
What are its climate targets?
Alongside its EU and Paris commitments, the UK Houses of Parliament approved the Climate Change Act in 2008, which commits to reducing GHG emissions by at least 80% of 1990 levels by 2050.
The Act requires the government to set legally-binding carbon budgets, a cap on the amount of GHG emitted in the UK over a five-year period. The first five carbon budgets have been put into legislation and will run up to 2032. These include reducing emissions 37% below 1990 levels by 2020 and 57% by 2030.
A key milestone in the UK’s decarbonisation is to entirely phase out coal by 2025, which will mean either closing or converting (as in the case of Drax Power Station) existing coal power stations.
How does it plan to achieve this?
Under its legally binding carbon budget system, every tonne of GHG emitted between now and 2050 will count. Where emissions rise in one sector of the economy (be it agriculture, heavy industry, power, transport, etc.), the UK must achieve corresponding falls in another.
The UK’s initial focus has been to transition to renewable electricity production. Wind, biomass and solar power have all grown significantly, aided by government support, and by initiatives like the carbon price floor.
How is it doing so far?
The UK’s progress towards its targets is positive, but leaves room for improvement. Renewables generated 14.9% of the UK’s electricity in 2013. In 2015 they accounted for nearly a quarter of electricity generation and by 2016, low carbon power sources contributed an average of 40% of the UK’s power, with wind generating more power than coal for the first time ever.
The Department for Business, Energy and Industrial Strategy estimates that as of 2016 GHG emissions fell 42% since 1990. Despite this, the Committee on Climate Change (CCC) has said that the government is not on track to meet its pledge of cutting emissions 80% by 2050.
However, it points out the UK is likely to meet the target of making electricity almost entirely low-carbon by early 2030s, but only if further steps are taken such as including increasing investment in more low-carbon generation (such as biomass), and developing carbon capture and storage (CCS) technologies. The UK government is due to publish an emissions reduction plan in the autumn of 2017.
Norway
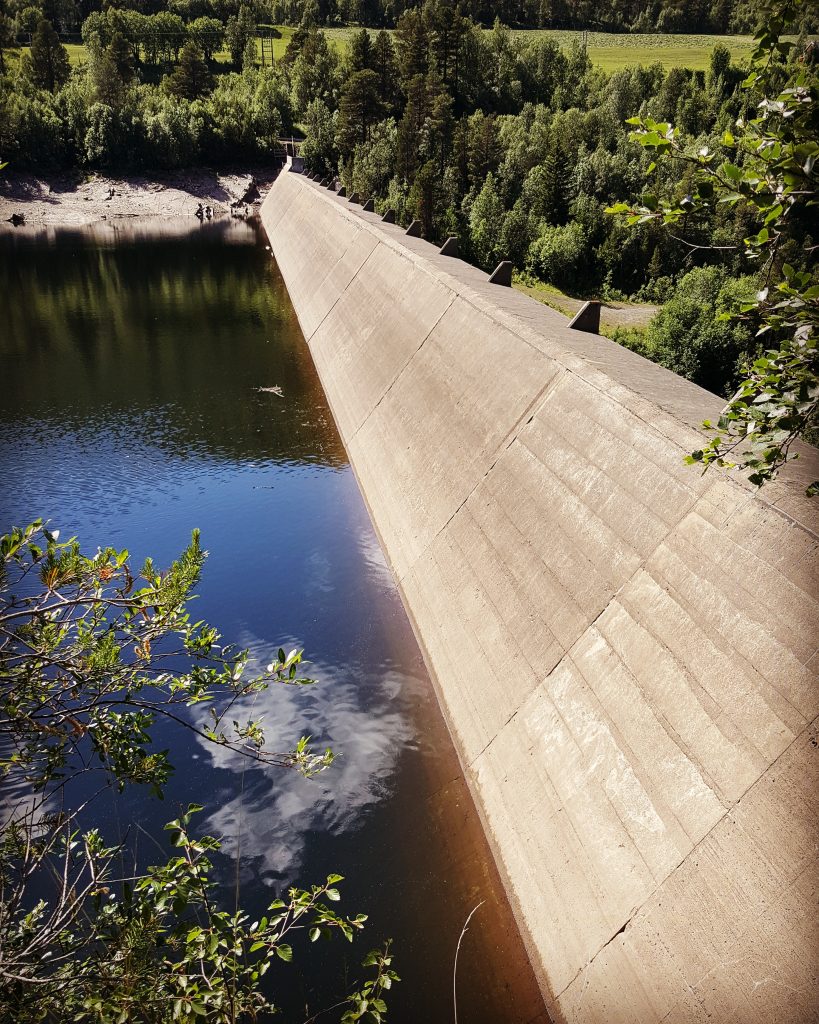
What are its climate targets?
Norway’s climate policy is based on agreements reached in the Storting (the Norwegian Parliament) in 2008 and 2012. They stipulate a commitment to reduce global GHG emissions by at least 30% by 2020 from 1990 levels. The government also approved the goal of achieving carbon neutrality by 2050.
As well as signing the Paris Agreement, Norway has aligned itself with the European Union’s climate target and intends to fulfil its commitment collectively with the EU (of which it is not a member state). This means using the EU emissions trading market, international cooperation on emissions reductions, and project-based cooperation.
How does it plan to achieve this?
Around 98% of Norway’s electricity production already comes from renewable energy sources, mostly through its more than 900 hydropower plants. The remainder is through wind and thermal power.
Norway exports hydropower to the Netherlands and exchanges renewable energy with Denmark, Sweden and Finland. There are plans for similar green exchanges with Germany and the United Kingdom via interconnectors within the next five years.
Norway is also aided by a substantial carbon sink in its forests which cover 30% of its land surface. They sequester (absorb and store) carbon from the atmosphere to such an extent that it equals approximately half of the Scandinavian country’s annual emissions.
How is it doing so far?
While Norway already has one of the world’s most carbon neutral electricity sectors, its significant domestic oil and gas sector means it still struggles to reduce its overall emissions. As such, the government is expected to rely on carbon trading with the EU or international offsets to meet its ambitious goals.
Nonetheless, earlier this year the government said that GHG emissions will fall to around 1990 levels by 2020, although it did not stipulate whether this included buying carbon credits from abroad or not.