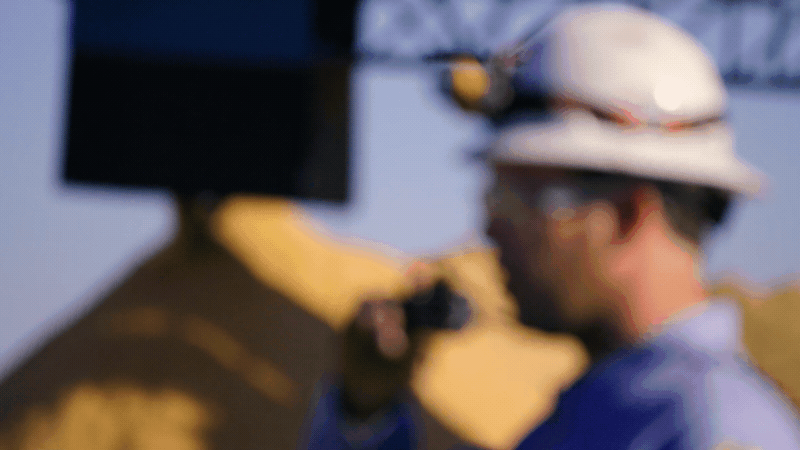
Find out about sustainable forest biomass
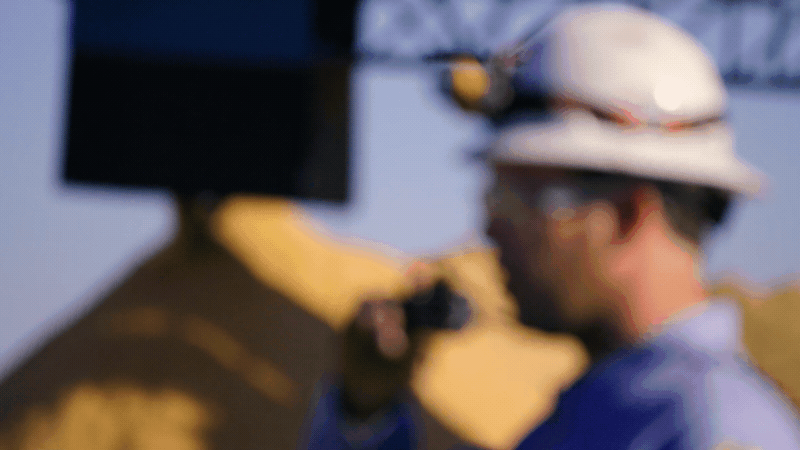
To tackle the climate crisis, global unity and collaboration are needed. This was in part the thinking behind the Paris Agreement. It set a clear, collective target negotiated at the 2015 United Nations Climate Change Conference and signed the following year: to keep the increase in global average temperatures to well below 2 degrees Celsius above pre-industrial levels.
In November 2021, COP26 will see many of the countries who first signed the Paris Agreement come together in Glasgow for the first ‘global stocktake’ of their environmental progress since its creation.
COP26 will take place at the SEC in Glasgow
Already delayed for a year as a result of the pandemic, COVID-19 and its effects on emissions is likely to be a key talking point. So too will progress towards not just the Paris Agreement goals but those of individual countries. Known as ‘National Determined Contributions’ (NDCs), these sit under the umbrella of the Paris Agreement goals and set out individual targets for individual countries.
With many countries still reeling from the effects of COVID-19, the question is: which countries are actually on track to meet them?
The NDCs of each country represent its efforts and goals to reduce national emissions and adapt to the impacts of climate change. These incorporate various targets, from decarbonisation and forestry to coastal preservation and financial aims.
While all countries need to reduce emissions to meet the Paris Agreement targets, not all have an equally sized task. The principle of differentiated responsibility acknowledges that countries have varying levels of emissions, capabilities and economic conditions.
The Universal Ecological Fund outlined the emissions breakdown of the top four emitters, showing that combined, they account for 56% of global greenhouse gas emissions. China is the largest emitter, responsible for 26.8%, followed by the US which contributes 13.1%. The European Union and its 28 member states account for nine per cent, while India is responsible for seven per cent of all emissions.
These nations have ambitious emissions goals, but are they on track to meet them?
Traffic jams in the rush hour in Shanghai Downtown, contribute to high emissions in China.
By 2030, China pledged to reach peak carbon dioxide (CO2), increase its non-fossil fuel share of energy supply to 20% and reduce the carbon intensity – the ratio between emissions of CO2 to the output of the economy – by 60% to 65% below 2005 levels.
COVID-19 has increased the uncertainty of the course of China’s emissions. Some projections show that emissions are likely to grow in the short term, before peaking and levelling out sometime between 2021 and 2025. However, according to the Climate Action Tracker it is also possible that China’s emissions have already peaked – specifically in 2019. China is expected to meet its non-fossil energy supply and carbon intensity pledges.
The forecast for the second largest emitter, the US, has also been affected by the pandemic. Economic firm Rhodium Group has predicted that the US could see its emissions drop between 20% and 27% by 2025, meeting its target of reducing emissions by 26% to 28% below 2005 levels.
However, President Trump’s rolling back of Obama-era climate policies and regulations, his support of fossil fuels and withdrawal from the Paris Agreement (effective from as early as 4 November 2020), suggest any achievement may not be long-lasting.
The United States’ Coronavirus Aid, Relief, and Economic Security Act, known as the CARES Act, does not include any direct support to clean energy development – something that could also change in 2021.
Carl Clayton, Head of BECCS at Drax, inspects pipework in the CCUS area of Drax Power Station
The European Union and its member states, then including the UK, pledged to reduce emissions by at least 40% below 1990 levels by 2030 – a target the Climate Action Tracker estimates will be achieved. In fact, the EU is on track to cut emissions by 58% by 2030.
This progress is in part a result of a large package of measures adopted in 2018. These accelerated the emissions reductions, including national coal phase-out plans, increasing renewable energy and energy efficiency. The package also introduced legally binding annual emission limits for each member state within which they can set individual targets to meet the common goal.
The UK has not yet released an updated, independent NDC. However, it has announced a £350 million package designed to cut emissions in heavy industry and drive economic recovery from COVID-19. This includes £139 million earmarked to scale up hydrogen production, as well as carbon capture and storage (CCS) technology, such as bioenergy with carbon capture (BECCS) – essential technologies in achieving net zero emissions by 2050 and protecting industrial regions.
India, the fourth largest global emitter, is set to meet its pledge to reduce its emissions intensity by 33% to 35% below 2005 levels and increase the non-fossil share of power generation to 40% by 2030. What’s more, the Central Electricity Agency has reported that 64% of India’s power could come from non-fossil fuel sources by 2030.
Wind turbines in Jaisalmer, Rajasthan, India
Along with increasingly renewable generation, the implementation of India’s National Smart Grid Mission aims to modernise and improve the efficiency of the country’s energy system.
It is promising that the world’s four largest emitters have plans in place and are making progress towards their decarbonisation goals. However, tackling climate change requires action from around the entire globe. In addition to NDCs, many countries have committed to, or have submitted statements of intent, to achieve net zero carbon emissions in the coming years.
Country | Target Date | Status |
---|---|---|
Bhutan 🇧🇹 | Currently carbon negative (and aiming for carbon neutrality as it develops; pledged towards the Paris Agreement) | |
Suriname 🇸🇷 | Currently carbon negative | |
Denmark 🇩🇰 | 2050 | In law |
France 🇫🇷 | 2050 | In law |
Germany 🇩🇪 | 2050 | In law |
Hungary 🇭🇺 | 2050 | In law |
New Zealand 🇳🇿 | 2050 | In law |
Scotland 🏴 | 2045 | In law |
Sweden 🇸🇪 | 2045 | In law |
United Kingdom 🇬🇧 | 2050 | In law |
Bulgaria 🇧🇬 | 2050 | Policy Position |
Canada 🇨🇦 | 2050 | Policy Position |
Chile 🇨🇱 | 2050 | In policy |
China 🇨🇳 | 2060 | Statement of intent |
Costa Rica 🇨🇷 | 2050 | Submitted to the UN |
EU 🇪🇺 | 2050 | Submitted to the UN |
Fiji 🇫🇯 | 2050 | Submitted to the UN |
Finland 🇫🇮 | 2035 | Coalition agreement |
Iceland 🇮🇸 | 2040 | Policy Position |
Ireland 🇮🇪 | 2050 | Coalition Agreement |
Japan 🇯🇵 | 2050 | Policy Position |
Marshall Islands 🇲🇭 | 2050 | Pledged towards the Paris Agreement |
Netherlands 🇳🇱 | 2050 | Policy Position |
Norway 🇳🇴 | 2050 in law, 2030 signal of intent | |
Portugal 🇵🇹 | 2050 | Policy Position |
Singapore 🇸🇬 | As soon as viable in the second half of the century | Submitted to the UN |
Slovakia 🇸🇰 | 2050 | Policy Position |
South Africa 🇿🇦 | 2050 | Policy Position |
South Korea 🇰🇷 | 2050 | Policy Position |
Spain 🇪🇸 | 2050 | Draft Law |
Switzerland 🇨🇭 | 2050 | Policy Position |
Uruguay 🇺🇾 | 2030 | Contribution to the Paris Agreement |
While the COVID-19 pandemic has disrupted short-term plans, many see it as an opportunity to rejuvenate economies with sustainability in mind. COP26, as well as the global climate summit planned for December of this year, will likely see many countries lay out decarbonisation goals that benefit both people’s lives and the planet.
#COP26, as well as the UK/UN climate meeting planned for this December, will likely see many countries lay out decarbonisation goals that benefit both people’s lives and the planet. #COP26countdown ⏰ #COP26Ambition 🌍
— Drax (@DraxGroup) October 30, 2020
The seventh report in a series of catchment area analyses for Drax looks at the fibre sourcing area surrounding a number of compressed wood pellet plants operated by Georgia Biomass (now owned by Enviva) and Fram Renewable Fuels.
The evidence found in the report by Hood Consulting shows a substantial increase in forest inventory (stored carbon) and a relatively stable forest area. However, with continued pressure from urban development, future losses of timberland area are possible. Despite this, increasing growth rates can maintain and improve wood supply and carbon stock for the foreseeable future.
The overall inventory of growing stock in the catchment area has increased by 63 million cubic metres (m3) between 2000 and 2018, a growth of 19.3%. All of this increase has been in the pine area, which increased by nearly 68 million m3, whereas the hardwood species decreased in volume by 4.5 million m3. Overall, the inventory volume split by species in 2018 was 72% to 28% softwood to hardwood. The breakdown by product category is shown in Figure 3 below.
Figure 1: Change in growing stock by major product category and species (USFS)
The pine saw-timber and chip-n-saw product categories, larger dimension and higher value material, showed the largest increase in inventory, whereas pine pulpwood decreased in total volume. The most substantial change occurred from 2010 to 2018, where pulpwood went from an increasing trend to a decreasing trend and saw-timber increased in volume much more rapidly – this is shown in Table 1 and Figure 2 below.
Change (cubic metres (m3)) | Pine Sawtimber | Pine Chip-n-saw | Pine Pulpwood | Hardwood Sawtimber | Hardwood Pulpwood | Total |
---|---|---|---|---|---|---|
2000-2018 | 51,301,628 | 22,277,139 | -5,835,230 | 1,211,110 | -5,657,114 | 63,297,533 |
2000-2010 | 14,722,995 | 12,707,674 | 5,262,192 | -3,740,507 | -5,769899 | 23,182,455 |
2010-2018 | 36,578,632 | 9,569,465 | -11,097,422 | 4,951,618 | 112,784 | 40,115078 |
These changes are likely to reflect an increasing age class in the catchment area, with younger stands of pine (previously classed as pulpwood), growing into a larger size class and being reclassified as saw-timber. This means that the volume of saw-timber availability in future will be significantly higher, but pulpwood availability will be diminished. For pellet mill markets any loss in pulpwood availability can be compensated by an increase in sawmill residue production if market demand is maintained or increased.
Figure 2: Change in growing stock by major product category and species (USFS)
Growth rates for both softwood and hardwood species have been increasing since 2000 as shown in Figure 3 below. Softwood growth has increased by 18.5% since 2000 and hardwood by 1.4%. The improved softwood growth rate probably resulted from increased investment in the management of pine forests, the superior quality of seedlings and better management practice (ground preparation, weed control, fertilisation etc.). This is a very positive trend for the sequestration rate of carbon and also for providing landowners with the potential to increase revenue per hectare and encourage the retention and improved management of forests, rather than converting to other land uses. The Georgia catchment area is likely split between passive owners that do not actively manage, where growth rates are slower or decline and the incentive to convert land is greater, and owners that actively manage to improve growth and quality, increasing revenue and maintaining productive forest. There is likely to be a much greater differential in growth rate between these two management approaches than reflected by the trend in Figure 3, highlighting the importance of active management for carbon abatement.
Figure 3: Average annual growth rate per hectare (USFS)
At a macro scale, the distribution of land use categories has remained relatively stable since 2000, with no apparent major shifts in land use. The timberland area around the seven mills has decreased by around 135 thousand hectares (ha) between 2000 and 2018 (2.3% of the total land area), whilst the area of arable and urban land increased by 98 thousand (1.7% of total area) and 158 thousand (2.7% of total area) ha respectively. In 2018, timberland represented 67% of total land area and all forest and woodland 80% of total area, down from 69% and 82% respectively in 2000 (Figure 1).
Figure 4: Change in land use category (USDA)
Looking at this change in land use more closely, the timberland area shows the most pronounced decline between 2010 and 2018, a drop of 117 thousand ha. The largest change in other land use categories over this period was an increase of 97 thousand ha in urban and other land, suggesting that a large proportion of the timberland area has been converted to urban areas.
The most significant change in agricultural land occurred prior to 2010, when the timberland area remained relatively stable, this change appears to have involved the transition of pastureland to arable crops. There may also have been some reclassification of forest and woodland types, with a decrease in the area of woodland and an increase in forestland during the period between 2000 and 2010 (Table 2).
Change (hectares (ha)) | Timberland | Other Forestland | Arable Cropland | Woodland | Pastureland | Urban & Other Land |
---|---|---|---|---|---|---|
2000-2018 | -135,195 | 70,073 | 98,436 | -77,904 | -113,725 | 178,315 |
2000-2010 | -18,539 | 53,150 | 73,243 | -73,077 | -95,630 | 60,852 |
2010-2018 | -116,656 | 16,922 | 25,193 | -4,827 | -18,096 | 97,463 |
These trends are also clear and apparent in Figure 3 below which shows the sharp decline in timberland area, albeit small in absolute area relative to the total catchment area size, and the steady increase in urban land. Georgia ranks 8th in the list of US States and territories by total population with 10.6 million and 17th by population density at 184 per square mile (mi2) compared to just 63 per mi2 in Mississippi where Drax’s Amite Bioenergy (ABE) pellet plant is located and 108 per mi2 in Louisiana where the Morehouse Bioenergy (MBE) and LaSalle Bioenergy (LBE) mills are located (US Census Bureau). This population pressure and increased development can lead to more forest loss and land use change.
Figure 5: Trends in major land use categories (USDA)
Drax’s suppliers in the Georgia catchment area have made a commitment not to source wood from areas where land use change is taking place. This commitment is monitored and verified through the Sustainable Biomass Program (SBP) certification process that is maintained by each mill. Any land use change in the catchment area is likely to be a result of prevailing economic drivers in the region rather than due to actions being taken by the pellet producers.
Strong markets are essential for ensuring that forests are managed and restocked to optimum benefit, sawlog markets are particularly important as this is highest revenue stream for forest owners. Figure 6 shows the trend in market demand for each major product category since 2000 and demonstrates the recent increase in softwood sawlog demand as the US economy (particularly housing starts) recovered from the global recession at the end of the last decade. Softwood pulpwood demand increased through the 2000s but has remained relatively stable since 2011, with the exception of a peak during 2018 which resulted from an increase in volume generated by salvage operations after hurricane Michael.
Figure 6: Demand for wood products (USFS, TMS)
The comparison of average annual growth and removals in the Georgia catchment area is much more tightly balance than in Drax’s other supply regions, as shown in Figure 7. Since 2000 the average annual surplus of growth has been around 3.6 million m3 with both demand and growth increasing in recent years.
Figure 7: Average annual growth, removals and surplus (USFS)
As shown in Figures 2 & 3, growth rates are strong and inventory is increasing, this is not a problem in the Georgia area. The relatively small surplus, as compared to other catchment areas in the US South, is due to the higher concentration of wood fibre markets and the more intense forest industry activity in this region. As of July 2020, there were over 50 major wood-consuming mills operating within the Georgia catchment area and an additional 80+ mills operating within close proximity, overlapping the catchment area. Total pulpwood demand in 2019 was 12.9 million tons, of which approximately 87% was attributed to non‐bioenergy‐related sources (predominantly pulp/paper) and 13% was attributed to the bioenergy sector. Given the bio-energy sector’s low ranking position in the market (with the lowest ability to pay for fibre), combined with the relatively small scale in demand compared to the pulp and paper industry, the influence of biomass markets can be considered to be minimal in this region, particular when it comes to impacts on wood prices and forest management practice.
Pine sawtimber prices suffered a significant decline between 2000 and 2010, dropping almost $21 per ton as a result of the global financial crisis and the decline in demand due to the collapse in housing markets and construction (Table 3). Since 2010 pine sawtimber has remained relatively stable, with some minor fluctuations shown in Figure 8 below.
Change ($/ton) | Pine Sawtimber | Pine Chip-n-saw | Pine Pulpwood | Hardwood Sawtimber | Hardwood Pulpwood |
---|---|---|---|---|---|
2000-2019 | -$20.92 | $15.14 | $5.95 | $12.55 | $4.70 |
2000-2010 | -$20.92 | -$21.41 | $2.11 | $11.25 | $5.67 |
2010-2019 | $0.00 | $6.27 | $3.84 | $1.30 | -$0.97 |
Pine pulpwood prices have been on a generally increasing trend since 2000, with a more significant increase since 2011. This increase does not reflect an increase in demand or total volume, which has remained relatively stable over this period, but a shifting of the geographic distribution of the market with some new mills opening and old mills closing, resulting in increased competition in some localised fibre baskets and leading to an overall increase in stumpage price.
Figure 8: Stumpage price trends (TMS)
Figure 9 below shows that, with the exception of the hurricane salvage volume in 2018, pulpwood removals have declined or remained relatively stable since 2010, whereas pulpwood stumpage prices increased by 41% from 2010 to 2018.
Figure 9: Pulpwood demand and stumpage price (USFS, TMS)
Comparing this stumpage price trend with other catchment areas of the US South (Figure 10), where Drax sources wood pellets, the Georgia area is on average 35% higher than the next highest area (Chesapeake) and 87% higher than the lowest cost area (Amite Bioenergy in Mississippi). This price differential is predominantly due to the scale of demand and availability of surplus low-grade fibre.
Figure 10: Comparison of pine pulpwood stumpage prices in Drax supply areas US South (TMS)
Deforestation?
No. US Forest Service (USFS) data shows a 108,130-hectare (-2.6%) decrease in total timberland in the Georgia catchment area since Georgia Biomass’ first full year of production in 2012. Specifically, this loss in total area of timberland coincided with a more than 21,000-hectare increase in cropland/pastureland and a more than 73,000-hectare increase in urban land and land classified as having other uses.
However, there is little evidence to suggest that increased wood demand from the bioenergy sector has caused this decrease in total timberland. Furthermore, pine timberland – the primary source of roundwood utilized by the bioenergy industry – has increased more than 17,000 hectares in the catchment area since 2016.
A change in management practices (rotation lengths, thinnings, conversion from hardwood to pine)?
No. Changes in management practices have occurred in the catchment area over the last two decades. However, there is little evidence to suggest that bioenergy demand, which accounts for roughly 10-14% of total pulpwood demand (and only 5-7% of total wood demand in the catchment area), has caused or is responsible for these changes.
Clearcuts and thinnings are the two major types of harvests that occur in this region, both of which are long-standing, widely used methods of harvesting timber. TimberMart-South (TMS) data shows that thinnings accounted for 67% of total reported harvest area in the southeast Georgia market from 2000-2010, but only 43% of total harvest area reported from 2012-2019. Specifically, this downward shift was initiated by the bursting of the US housing bubble in the mid-2000s and had been completed by the early 2010s. We’d like to note that this shift coincided with a nearly 50% decrease in pine sawtimber stumpage price from 2006-2012. This is important because the strength of pine sawtimber markets had been a driving force behind timber management decisions in this region in the early and mid-2000s.
Also, contributing to the decreased prevalence of thinnings was the strengthening of pine pulpwood markets in the mid-2000s, as pine pulpwood stumpage prices increased more than 40% in the Georgia catchment area from 2003-2008. So, with sawtimber markets continuing to weaken and pulpwood markets doing just the opposite, the data suggests that many landowners decided to alter their management approach (to take advantage of strong pulpwood markets) and focus on short pulpwood rotations that typically do not utilize thinnings.
Ultimately, the shift in management approach that occurred in this market can be linked to the weakening of one type of timber market and the strengthening of another. In the early and mid-2000s, timber management was focused on sawtimber production – a type of management that utilizes thinnings. However, for more than a decade now, this market has been driven to a large degree by the pulp/paper industry, with a significant portion of the timber management in this area focused on short pulpwood rotations.
Diversion from other markets?
No. Demand for softwood (pine) sawlogs increased an estimated 39% in the Georgia catchment area from 2011-2019. Also, increased bioenergy demand has caused no diversion from other pulpwood markets (i.e. pulp/paper), as pulpwood demand not attributed to bioenergy held steady and remained nearly unchanged from 2012-2017 before increasing in 2018 and 2019 due to the influx of salvage wood brought about by Hurricane Michael.
We’d like to make special note that increased demand for softwood sawlogs since 2011 has not resulted in a full pine sawtimber (PST) stumpage price recovery in this market. Reduced demand for softwood sawlogs in the late 2000s and early 2010s resulted in oversupply, and this oversupply has remained, despite increased demand the last 6-8 years. As a result, PST stumpage prices have held steady and averaged roughly $30 per ton in the catchment area since 2013 – down approximately 35% from the 2000-2006 average of more than $46 per ton, but up roughly 15% from the 2011-2012 average of approximately $26 per ton.
An unexpected or abnormal increase in wood prices?
No / Inconclusive. The delivered price of pine pulpwood (PPW) – the primary roundwood product consumed by both Georgia Biomass and Fram – increased 26% in the Georgia catchment area over the six years directly following the startup of Georgia Biomass, increasing from $29.16 per ton in 2011 to $36.63 per ton in 2017. And while this 26% increase in delivered PPW price coincided with a roughly 1.1 million metric ton increase in annual pine pulpwood demand from Georgia Biomass and Fram, total demand for pine pulpwood (from both bioenergy and other sources) actually decreased 7% over this period. Moreover, evidence suggest that this increase in PPW price is more closely linked to changes in wood supply, specifically, the 9% decrease in PPW inventory from 2011-2017.
However, there is evidence that links increased demand from the bioenergy sector to an increase in secondary residual (i.e. sawmill chips, sawdust, and shavings) prices. Specifically, the price of pine sawmill chips – a residual feedstock utilized by the bioenergy industry for wood pellet production – held steady and averaged approximately $26 per ton in the Georgia catchment area from 2008-2012. However, from 2012-2016, pine sawmill chip prices increased more than 15% (to $29.55 per ton in 2016). This increase in price coincided with annual pine residual feedstock purchases by Georgia Biomass and Fram increasing from roughly 325,000 metric tons to nearly 1.0 million metric tons over this period. However, note that pine sawmill chip prices have held steady and averaged roughly $29.50 per ton in the catchment area since 2016, despite further increases in pine secondary residual purchases by Georgia Biomass and Fram (to more than 1.2 million metric tons in 2019).
Ultimately, the data suggests that any excess supply of pine secondary residuals in the catchment area was absorbed by the bioenergy sector in the early and mid-2010s, and the additional demand/competition placed on this market led to increased residual prices. However, the plateauing of residual prices since 2015 along with the continued increase in secondary residual purchases by Georgia Biomass and Fram further suggest that an increasing percentage of secondary residual purchases by the bioenergy sector is sourced from outside the catchment area. Specifically, Fram confirmed this notion, noting that 35-40% of its secondary residual purchases come from outside the Georgia catchment area (from six different states in the US South).
A reduction in growing stock timber?
No. Total growing stock inventory in the catchment area increased 11% from 2011 through 2018, the latest available. Specifically, over this period, inventories of pine sawtimber and chip-n-saw increased 35% and 13%, respectively. However, pine pulpwood inventory decreased 11% from 2011-2018.
Note that the decrease in pine pulpwood inventory was not due to increased demand from bioenergy (or other sources) or increased harvesting above the sustainable yield capacity of the forest area – as annual growth of pine pulpwood has exceeded annual removals every year since 2011. Rather, this decrease can be linked to the 24% decline in pine sawtimber removals that occurred from 2005-2014 (due to the bursting of the US housing bubble and Great Recession that followed). In this region, timber is typically harvested via clearcut once it reaches maturity (i.e. sawtimber grade), after which the stand is reestablished, and the cycle repeated. However, with the reduced harvest levels during this period also came a reduction in newly reestablished timber stands – the source of pine pulpwood. So, with less replantings occurring during this period, inventories of pine pulpwood were not replenished to the same degree they had been previously, and therefore this catchment area saw a reduction in pine pulpwood inventory levels.
However, according to the US Forest Service, annual removals of pine sawtimber have increased 50% in the Georgia catchment area since 2014, which would suggest higher clearcut levels and increased stand reestablishment. TimberMart-South data also supports this assertion, as clearcut harvests have constituted approximately 60% of the total harvest area reported to TimberMart-South in this region since 2014, compared to 40% from 2005-2014. Ultimately, these increases in clearcut (and stand reestablishment) levels may not be reflected in increased pine pulpwood inventory levels in the short term – as it can take more than 10 years for a pine seedling to become merchantable and reach the minimum diameter requirements to be classified as pulpwood. However, adequate supply levels are expected to remain in the meantime. Furthermore, pine pulpwood inventory levels are expected to increase in the mid-to-long terms as a result of the increased harvest levels and stand reestablishment levels that have occurred in the catchment area since 2014.
A reduction in the sequestration rate of carbon?
No / Inconclusive. US Forest Service data shows the average annual growth rate of total growing stock timber has remained nearly unchanged (holding between 6.0% and 6.1%) in the catchment area since 2011, which would suggest that the sequestration rate of carbon has also changed very little in the catchment area the last 8-10 years. However, the 11% increase in total growing stock inventory since 2011 does indicate that total carbon storage levels have increased in the Georgia catchment area since Georgia Biomass commenced operations in this market.
An increase in harvesting above the sustainable yield capacity of the forest area?
No. Growth-to-removals (G:R) ratios, which compare annual timber growth to annual harvests, provides a measure of market demand relative to supply as well as a gauge of market sustainability. In 2018, the latest available, the G:R ratio for pine pulpwood, the predominant timber product utilized by the bioenergy sector, equaled 1.06 (a value greater than 1.0 indicates sustainable harvest levels). Note, however, that the pine pulpwood G:R ratio averaged 1.44 from 2012-2017. The significant drop in 2018 was due to a 31% increase in removals (due to Hurricane Michael) and is not reflective of the new norm. Specifically, pine pulpwood removals are projected to be more in line with pre-2018 levels in 2019 and 2020, and so too is the pine pulpwood G:R ratio.
Timber growing stock inventory
Neutral. According to USFS data, inventories of pine pulpwood decreased 11% in the catchment area from 2011-2018. However, that decrease was not due to increased demand from bioenergy. Typically, a reduction in inventory is linked to harvest levels above the sustainable yield capacity of the forest area, but in this case, annual growth of pine pulpwood exceeded annual removals every year during this period.
Ultimately, the decrease in pine pulpwood inventory from 2011-2018 can be linked to decreased pine sawtimber production beginning in the mid-2000s. Specifically, annual removals of pine sawtimber decreased 24% from 2005-2014, and the reduction in harvest levels during this period meant fewer new pine stands were reestablished, and that has led to the current reduction in pine pulpwood inventory. (Note that the decrease in pine sawtimber removals from 2005-2014 was mirrored by a 27% increase in pine sawtimber inventory over this same period). However, USFS data shows that annual removals of pine sawtimber have increased 50% in the Georgia catchment area since 2014, which suggests that pine pulpwood inventory levels will start to increase in the catchment area due to increased harvest levels and the subsequent increase in stand reestablishment levels.
Timber growth rates
Neutral. Timber growth rates have increased for both pine sawtimber and pine chip-n-saw but decreased slightly for pine pulpwood in the catchment area since 2011. Evidence suggests that this decrease in pine pulpwood growth rate is not due to increases in bioenergy demand, but rather linked to changes in diameter class distribution and indicative of a forest in a state of transition, where timber is moving up in product class (i.e. pine pulpwood is moving up in classification to pine chip-n-saw).
Forest area
Neutral. In the Georgia catchment area, total forest area (timberland) decreased more than 115,000 hectares (-2.8%) from 2011 through 2018. Note that this decrease coincided with a roughly 19,000-hectare increase in cropland and 93,000-hectare increase in urban land and land classified as having other uses.
Specifically, pine timberland, the primary source of roundwood utilized by the bioenergy industry, decreased over 34,000 hectares from 2011-2016. However, from 2016-2018, pine timberland stabilized and rather increased more than 17,000 hectares in the catchment area (or a net decrease of roughly 17,000 hectares from 2011-2018). Ultimately, there is little evidence that the decrease in pine timberland from 2011-2016 or increase since 2016 is linked to increased bioenergy demand. Rather, the overall decrease in pine timberland since 2011 appears to be more closely linked to the relative weakness of pine sawtimber markets in the Georgia catchment area and the lack of return from sawtimber.
Wood prices
Positive / Negative. Intuitively, an increase in demand should result in an increase in price, and this is what the data shows in the Georgia catchment area as it relates to increased biomass demand from Georgia Biomass and Fram and the prices of the various raw materials consumed by these mills. Specifically, the 1.4-million metric ton increase in softwood pulpwood demand attributed to Georgia Biomass and Fram coincided with a 20% increase in delivered pine pulpwood price and a 10-15% increase in pine chip prices from 2011-2015. Since 2015, biomass demand has held relatively steady, and, overall, so too have delivered pine pulpwood and pine chip prices. The apparent link between increased bioenergy demand and increased pine raw material prices is supported further by statistical analysis, as strong positive correlations were found between softwood biomass demand and both delivered pine pulpwood and pine chip prices. However, note that biomass demand alone is not responsible for these changes in prices, as softwood biomass demand accounts for only 10-15% of total softwood pulpwood demand in the catchment area. Rather, the prices of these raw materials are impacted to a larger degree by demand from other sources (i.e. pulp/paper), which accounts for 85-90% of total softwood pulpwood demand in the Georgia catchment area.
On the other hand, it’s also important to note that the increase in bioenergy-related wood demand has been a positive for forest landowners in the Georgia catchment area. Not only has bioenergy provided an additional outlet for pulpwood in this market, but the increase in pulpwood prices as a result of increased pulpwood demand has transferred through to landowners (improved compensation). Specifically, since 2015, pine pulpwood (PPW) stumpage price – the price paid to landowners – has averaged more than $17 per ton in the Georgia catchment area. This represents a 70% increase over the approximately $10 per ton averaged by PPW stumpage in the catchment area over the last five years prior to Georgia Biomass’ startup in 2Q 2011.
(Note: Pine pulpwood stumpage prices are notably higher in the Georgia catchment area due to a much tighter balance in supply and demand (in comparison to most other markets across the US South). For instance, in all other areas across the US South2, PPW stumpage prices have averaged less than $9 per ton since 2015, or roughly half that of prices in the Georgia catchment area).
Markets for solid wood products
Positive. In the Georgia catchment area, demand for softwood sawlogs used to produce lumber and other solid wood products increased an estimated 39% from 2011-2019, and by-products of the sawmilling process are sawmill residuals – materials utilized by Georgia Biomass and the Fram mills to produce wood pellets. With the increased production of softwood lumber, so too has come an increase in sawmill residuals, some of which have been purchased/consumed by Georgia Biomass and Fram. Not only have these pellet producers benefited from the greater availability of this by-product, but lumber producers have also benefited, as the Georgia Biomass and Fram mills have provided an additional outlet for these producers and their by-products.
In ecological terms, biomass refers to any type of organic matter. When it comes to energy, biomass is any organic matter that can be used to generate energy, for example wood, forest residues or plant materials.
Biomass used and combusted for energy can come in a number of different forms, ranging from compressed wood pellets – which are used in power stations that have upgraded from coal – to biogas and biofuels, a liquid fuel that can be used to replace fossil fuels in transport.
The term biomass also refers to any type of organic material used for energy in domestic settings, for example wood burned in wood stoves and wood pellets used in domestic biomass boilers.
Biomass is organic matter like wood, forest residues or plant material, that is used to generate energy.
Biomass can be produced from different sources including agricultural or forestry residues, dedicated energy crops or waste products such as uneaten food.
Drax Power Station uses compressed wood pellets sourced from sustainably managed working forests in the US, Canada, Europe and Brazil, and are largely made up of low-grade wood produced as a byproduct of the production and processing of higher value wood products, like lumber and furniture.
Biomass producers and users must meet a range of stringent measures for their biomass to be certified as sustainable and responsibly sourced.
Biomass grown through sustainable means is classified as a renewable source of energy because of the process of its growth. As biomass comes from organic, living matter, it grows naturally, absorbing carbon dioxide (CO2) from the atmosphere in the process.
It means when biomass is combusted as a source of energy – for example for heat or electricity production – the CO2 released is offset by the amount of CO2 it absorbed from the atmosphere while it was growing.
Biomass is a renewable, sustainable form of energy used around the world.
Biomass has been used as a source of energy for as long as humans have been creating fire. Early humans using wood, plants or animal dung to make fire were all creating biomass energy.
Today biomass in the form of wood and wood products remains a widely used energy source for many countries around the world – both for domestic consumption and at grid scale through power stations, where it’s often used to replace fossil fuels with much higher lifecycle carbon emissions.
Drax Power Station has been using compressed wood pellets (a form of biomass) since 2003, when it began research and development work co-firing it with coal. It fully converted its first full generating unit to run only on compressed wood pellets in 2013, lowering the carbon footprint of the electricity it produced by more than 80% across the renewable fuel’s lifecycle. Today the power station runs mostly on sustainable biomass.
Reforestation is the process of planting trees in a forest where the number of trees has been decreasing.
Afforestation is when new trees are planted or seeds are sown in an area where there were no trees before, creating a new forest.
Reforestation and afforestation are two of the leading nature-based solutions for tackling the effects of climate change. For commercial foresters and landowners, these two practices are essential to ensuring they can grow wood for wood products and continuously meet demand in a sustainable way.
Reforestation is crucial in combating or preventing deforestation or forest degradation, where forests shrink in size or are completely removed. As well as reducing a forest’s ability to absorb carbon dioxide (CO2), deforestation can destroy wildlife habitats and contribute to the likelihood of flooding in certain areas.
Afforestation can also help avoid desertification, where fertile land turns into a desert as a result of drought or intensive agriculture.
Reforestation is the process of planting native trees in a forest where the number of trees has been decreasing.
Forests are a natural way of keeping the earth’s CO2 levels in check. The more trees there are, the more CO2 is captured and converted into oxygen through photosynthesis.
By absorbing CO2, forests help to lower the amount of greenhouse gasses in the atmosphere and reduce the effects of climate change.
Reforestation and afforestation help maximize these abilities of forests by increasing the overall amount of forested land on the planet.
Different types of forests, such as tropical, swamps or mangroves, all absorb CO2 at different rates.
The age of a forest also impacts absorption. Young, rapidly growing, trees absorb CO2 at a faster rate than more mature ones, which have large amounts of carbon locked in already.
Afforestation is when new trees are planted or seeds are sown in an area where there were no trees before.
The global wood products industry depends on sustainable forests to supply the wood needed to make furniture, create construction materials and provide fuel for energy.
The supply chain will often start with what’s called a ‘working forest’ – a commercially-run forest which is often privately owned. The landowner will grow a working forest to a certain stage of maturity and then harvest some or all of the trees to sell the wood. Once the wood has been sold for use as lumber, wood products or fuel, the landowner will reforest the areas to regrow the trees.
Foresters will typically do this in stages across their land to ensure there are multiple stands of forest at different stages of growth across their land, which ensures there is consistent, sustainable growth at all times.
Scientific research isn’t all test tubes and lab coats – sometimes it’s bark and soil. It might be a world away from the image of a sterile laboratory, but the world of forestry is one that has seen significant scientific progress since the 18th century, when it first emerged as an area of study.
The development of environmental sciences and ecology, as well as advances in biology and chemistry mean there are still new discoveries being made – from trees’ ability to ‘talk’ to each other through underground fungi networks, to forests’ positive impact on mental health.
Fostering greater awareness and understanding of fragile forest ecosystems such as the cypress swamps of the Atchafalaya Basin in Louisiana, forestry has also allowed for the improvement of working forests — landscapes planted to grow wood for products and services that often avoid the use of fossil fuel-based alternatives.
Cypress forests in the Atchafalaya Basin in Louisiana are an example of a forest landscape where the suitable management practice is protection, preservation and monitoring
By enhancing the genetic stock, tree breeding ensures seedlings and plants are better adapted to their environment (soil, water, temperature, nutrient level, etc.). Science can now help trees to grow more quickly, storing more carbon. It can also give trees better form — straighter trees can produce more saw-timber which can, in turn, lock more carbon in buildings made predominantly or partially of the natural, renewable product that wood is.
But more than just uncovering surprising insights into the ins and outs of our natural world, forestry science is contributing to a far bigger goal: tackling climate change.
When the scientific study of forests first emerged in 18th century Germany, it was with the aim of sustainability in mind. Industries were concerned forests wouldn’t be able to provide enough timber to meet demand, so research began into how to manage them responsibly.
Forestry today encompasses much more than just providing saw logs and the research going into it remains driven by the same goal: to ensure sustainability. Its breadth, however, has grown.
The UK Forestry Commission’s research and innovation strategy highlights the scope it should cover: “It must be forward-looking to anticipate long-term challenges, strategic to inform emerging policy issues, and technical to support new and more efficient forestry practices.”
Pine trees grown for planting in the forests of the US South where more carbon is stored and more wood inventory is grown each year than fibre is extracted for wood products such as biomass pellets
Being able to deliver on this breadth has relied on rapid advances in technology – including taking forestry research into space.
As in almost every industry, one of the major drivers of change in forestry is data, and the ability to collect data from forests is getting more advanced.
At ground level, techniques like ‘sonic tomography’ allow foresters and researchers to ‘see’ inside trees using sound waves, measuring size, decay and overall health. This, in turn, offers a bigger picture of forests’ wellbeing.
At the other end of the scale, satellites and mapping technology are playing a major role in advancing a macro view of the world’s forests – particularly in how they change over time. As well as a potent tool in monitoring and helping fight deforestation, satellite images have revealed there is nine per cent more forest on earth than previously thought.
Space satellite with antenna and solar panels in space against the background of the earth. Image furnished by NASA.
The European Space Agency’s Earth Explorer programme will go a step further and use radar from satellites to penetrate the forest canopy, measuring tree trunks and branches rather than just the area covered by forest. Determining the volume of wood in forests around the planet will effectively enable researchers to ‘weigh’ the world’s forest biomass.
The masses of data these advances in tech are providing, is playing a major role in how we manage our forests, including how we can use them to fight global warming.
Forests are one of the key defences against climate change – so much so they’re included in the Paris Agreement. Trees’ abilities to absorb carbon dioxide (CO2) has long been established knowledge. Thanks to what climate scientists call IAMs or integrated assessment models, we now know how much they can extract from the atmosphere and how long they can continue to do so, as CO2 levels rise.
One optimistic hypothesis says trees will take in more CO2, as the levels rise. To test this, researchers in the UK are blasting controlled sections of a forest with CO2 to increase its density by 40%, representing expected global levels by 2050. By tracking how trees react they hope to highlight the role they can play as carbon sinks.
Science also suggests they could not only help slow climate change, but actively fight it. The research considers that as well as absorbing CO2, trees are reported to emit gases that reflect sunlight back into space, ultimately contributing to global cooling.
However, planting more trees isn’t necessarily the only answer. In places experiencing drought such as the western US, thinning forests can reduce competition and allow healthier trees capable of absorbing more oxygen to flourish.
The increasing body of research on forests’ impact on climate change could prove vital in shaping both the forestry industry and national governments’ approaches forests. However, as a science, forestry could be considered to be in its infancy. At this crucial time for the planet’s future, forestry is becoming one of the most important environmental sciences, but a lot more attention, investment and research and development are required if we are able to fully understand and manage the world’s forest resources. We have barely scratched the surface.
The wood supply catchment area for Drax’s LaSalle BioEnergy biomass pellet plant in mid-Louisiana is dominated by larger scale private forest owners that actively manage and invest in their forest for saw-timber production. Eighty-three per cent (83%) of the forest is in private ownership and 60% of this area is in corporate ownership.
The Drax Biomass pellet mill uses just 3.2% of the roundwood in the market and therefore has limited impact or influence on the overall trends. By contrast, the pulp and paper industry consumes 74% of the total pulpwood demand as the most dominant market for low grade fibre.
Forest in LaSalle catchment area
The catchment area has seen an increase in total timberland area of 71 thousand hectares (ha) since 2008, this is primarily due to planting of previously non-stocked land. Hardwood areas have remained stable but planted pine has increased, replacing some of the naturally regenerated mixed species areas. The data below shows that deforestation or conversion from pure hardwood to pine is not occurring.
Timberland area by management type
The overall quantity of stored carbon, or the inventory of the standing wood in the forest, has increased by 7% or 32.6 million metric tonnes since 2008. This total is made up of a 49 million tonne increase in the quantity of pine and a 16 million tonne decline in the quantity of hardwood. Since the area of pure hardwood forest has remained stable, this decline is likely to be due to the conversion of mixed stands to pure pine in order to increase saw-timber production and to provide a better return on investment for corporate owners.
Historic area and timberland inventory
Forest in LaSalle catchment area
The growth-to-drain ratio and the surplus of unharvested pine growth has been increasing year-on-year from two million tonnes in 2008 to over five million tonnes in 2016.
This suggests that the LaSalle BioEnergy plant (which almost exclusively utilises pine feedstocks) has not had a negative impact on the growth-to-drain ratio and the surplus of available biomass.
The latest data (2016) indicates that the ratio for pine pulpwood is 1.54 and for pine saw-timber 1.24 and that this has been increasing each year for both categories.
Historic growth and removals by species
Stumpage prices for all product categories declined between 2010 and 2011. This was followed by a peak around 2015-16 with the recovery in demand post-recession and prices then stabilised from 2016 to 2019. The data indicates that there has been no adverse impact to pine pulpwood prices as a result of biomass demand. In fact, pine pulpwood prices are now nearly 20% lower than in 2014 as shown on the chart below.
LaSalle BioEnergy market historic stumpage prices, USD$:tonne
The character of the pine timberland is one of a maturing resource, increasing in the average size of each tree. The chart below chart shows a significant increase in the quantity of timber in the mid-range size classes, indicating a build-up of future resources for harvesting for both thinning and final felling for sawtimber production.
With balanced market demand, the supply of fibre in this catchment area should remain plentiful and sustainable in the medium term.
Historic pine inventory by DBH (diameter at breast height) class
Deforestation
No
Change in forest management practices
No
Diversion from other markets
Possibly. Bioenergy plants compete with pulp/paper and oriented strand board (OSB) mills for pulpwood and residual feedstocks. There is no evidence that these facilities reduced production as a result of bioenergy markets, however.
Increase in wood prices
No. There is no evidence that bioenergy demand increased stumpage prices in the market.
Reduction in growing stock of timber
No
Reduction in sequestration of carbon / growth rate
No
Increase in harvesting above the sustainable yield
No
Growing stock
Neutral
Growth rates
Neutral
Forest area
Neutral
Wood prices
Neutral
Markets for solid wood
Neutral to Positive. Access to viable residual markets benefits users of solid wood (i.e. lumber producers).
Forest in LaSalle catchment area
There is an ongoing debate about forests’ contribution to fighting the climate crisis.
Forests can act as substantial and effective tools for carbon sequestration during a high growth phase. They can also function as significant and extensive carbon storage areas during maturity and throughout multiple stages of the age class cycle, if managed effectively at a landscape level. Or, they can be emitters of carbon if over-harvested, subject to fire, storm, pest or disease damage.
Different age class forest stands in Louisiana
In a natural state, forests will go through each of these life phases: rapid early growth; maturity and senescence; damage, decay and destruction through natural causes. Then they begin the cycle again, absorbing and then emitting carbon dioxide (CO2) in a continual succession.
Recently, loud voices have argued against forest management per se; against harvesting for wood products in particular, suggesting that this reduces both forest carbon stocks and sequestration capacity.
Pine cut in into wood for different wood products markets in Louisiana. Big, thick, straight higher value sections go to sawmills and smaller and misshapen low-grade wood not suitable for timber production is sold to pulp, paper or wood pellet mills.
Many foresters consider that this is just not correct. In fact, the opposite is true. Research and evidence clearly support the foresters’ view. Active forest management, when carried out appropriately, actually increases the amount of carbon sequestered, ensures that carbon is stored in solid wood products, and provides substantial savings of fossil fuels by displacing other high carbon materials (e.g. concrete, steel, brick, plastic and coal).
Oliver et al.(2014)[1] compared the impact of forest harvesting and the use of wood products to substitute other high-carbon materials, concluding that: ‘More CO2 can be sequestered synergistically in the products or wood energy and landscape together than in the unharvested landscape. Harvesting sustainably at an optimum stand age will sequester more carbon in the combined products, wood energy, and forest than harvesting sustainably at other ages.’
This research demonstrated that an increase in the use of structural timber to displace concrete and steel could lead to substantial emissions savings compared to unharvested forest. The use of wood for energy is an essential component of this displacement process, although it is important to use appropriate feedstocks. Burning wood that could be used for structural timber will not lead to a positive climate impact.
The message here is to manage working forests for optimum sawlog production for long-life solid wood products and utilise the by-products for energy where this is the most viable market, this provides the best all-round climate benefit.
Closing the forest gate and stopping all harvesting and management is one option being championed by some climate change campaigners. There is certainly a vital role for the preservation and protection of forests globally: primary and virgin forests, intact landscapes, high biodiversity and high conservation value areas all need to be protected.
That doesn’t necessarily mean that there is no forest management. It should mean careful and appropriate management to maintain and ensure the future of the resource. In these cases, management is with an objective to reduce the risk of fire, pests and disease, rather than for timber production.
Globally, we need better governance, understanding and implementation of best practice to achieve this. Forest certification and timber tracing systems are a good start. This can equally apply to the many hundreds of millions of hectares of ‘working forest’ that do not fall into the protection categories; forests that have been managed for many hundreds of years for timber production and other purposes. Harvesting in these forests can be more active, but governance, controls and the development of best practice are required. Better management not less management.
During the 1970s there was a significant change of policy in the US, aimed at removing massive areas of publicly owned forest from active management – effectively closing the gate. The drivers behind this policy were well meaning; it was intended to protect and preserve the habitat of endangered species, but the unintended consequences have also had a substantial impact. In the 1970s little thought was given to the carbon sequestration and storage potential of forests and climate change was not at the top of the agenda.
The west coast of the US was most substantially affected by these changes, more than in the US South, but the data below looks at the example of Mississippi which is primarily ‘working forest’ and 88% in private ownership.
Pine trees in Mississippi working forest
This is the location of Drax’s Amite pellet mill. The charts below show an interesting comparison of forest ownership in Mississippi where limited or no harvesting takes place and where active management for timber production occurs. In the short term the total volume of timber stored per hectare is higher where no harvesting occurs. This makes sense since the forest will keep growing until it reaches its climax point and succumbs to fire, pest or disease.
Average standing volume per unit area in the private sector, where active management occurs, is the lowest as timber is periodically removed for use in solid wood products. Remember that the Oliver et al. analysis (which does not include re-growth), showed that despite a short-term reduction in forest carbon, the total displacement of high-carbon materials with wood for structural timber and energy leads to a far higher emissions saving. It is better to have a lower stock of carbon in a working forest and to be continually sequestering new carbon for storage in solid wood products.
Average standing volume per acre by ownership class, Mississippi[2]
Average growth rates per acre by ownership class, Mississippi[3]
We know that forests are not being ‘lost’ and that the overall storage of carbon is increasing. For example, the Drax catchment area analysis for the Amite biomass wood pellet plant showed an increase in forest area of 5,200 ha and an increase in volume of 11 million m3 – just in the area around the pellet mill. But what happens to protected forest area, the forest reserve with limited or no harvesting?
Over the last 20 years the average annual loss of forest to wildfire in the US has been 2.78 million ha per year (the same as the UK’s total area of productive forest). According to the USFS FIA database the average standing volume of forests in the US is 145 m3 per ha (although in the National Park land this is 365 m3 per ha). Therefore, wildfires are responsible for the average annual combustion of 403 million m3 of wood p.a. (equal to the total annual wood harvest of the US) or 2.5 billion m3 if entirely in National Parks.
One cubic metre equates to a similar quantity of CO2 released into the atmosphere each year, therefore wildfires are responsible for between 407 million and 2.5 billion tonnes of CO2 emissions in the US each year[4].
Starrs et al. (2018)[5] demonstrated that the risk of wildfire was significantly higher in federally owned reserved forest (where harvesting and management were restricted), compared to privately owned forests with active management.
In California, the risk of wildfire in federal forest (2000-15) was almost double the risk in private forests where both had State firefighting resources. The risk of fires in federal lands had increased by 93% since 1950-66, compared to only 33% in non-federal forests, due to the change in forest management practice in the 1970s.
Forest fire in California
Closing the gate means that the carbon stock is maintained and grows in the short term, but there is no opportunity for carbon to be stored in solid wood products, no high-carbon materials are displaced (concrete, steel and fossil fuels) and the rate of sequestration declines as the forest ages. Eventually the forest will reach its natural climax and die, releasing all of that carbon back into the atmosphere. The managed forest, by contrast, will have a lower standing volume at a certain point in time, but will be in a continual cycle of sequestration, storage and regrowth – with a much lower risk of fire and disease. If managed correctly, the rate of growth and standing volume will also increase over time.
Forests are extremely variable, there are a vast variety of tree species, soil, geological features, water regimes, temperature, climate and many other factors that combine to make unique ecosystems and forest landscapes. Some of these are rare and valuable for the exceptional assemblages they contain, some are commonplace and widespread. Some are natural, some man-made or influenced by human activity.
Forests have many important roles to play and careful management is required. In some cases that management may be protection, preservation and monitoring. In other cases, it may be active harvesting and planting to optimise growth and carbon storage.
Cypress forests in the Atchafalaya Basin in Louisiana are an example of a forest landscape where the suitable management practice is protection, preservation and monitoring
For each forest type and area, we need to recognise the highest or best purpose(s) for that land in the objectives set and carefully plan the management to optimise and sustain that value. The primary value could be in species and habitat diversity or rarity; provision of recreation and aesthetic value; production of timber, forest products and revenue generation; carbon sequestration and storage; water management and other ecosystem benefits.
Most likely it will be a combination of several of these benefits. Therefore, best management practice usually involves optimising each piece of forest land to provide the most effective combination of values. Forests can deliver many benefits if we are sensible about how we manage them.
In a recent study Favero et al. (2020)[6] concluded that: Increased bioenergy demand increases forest carbon stocks thanks to afforestation activities and more intensive management relative to a no-bioenergy case. Some natural forests, however, are converted to more intensive management, with potential biodiversity losses…the expanded use of wood for bioenergy will result in net carbon benefits, but an efficient policy also needs to regulate forest carbon sequestration.