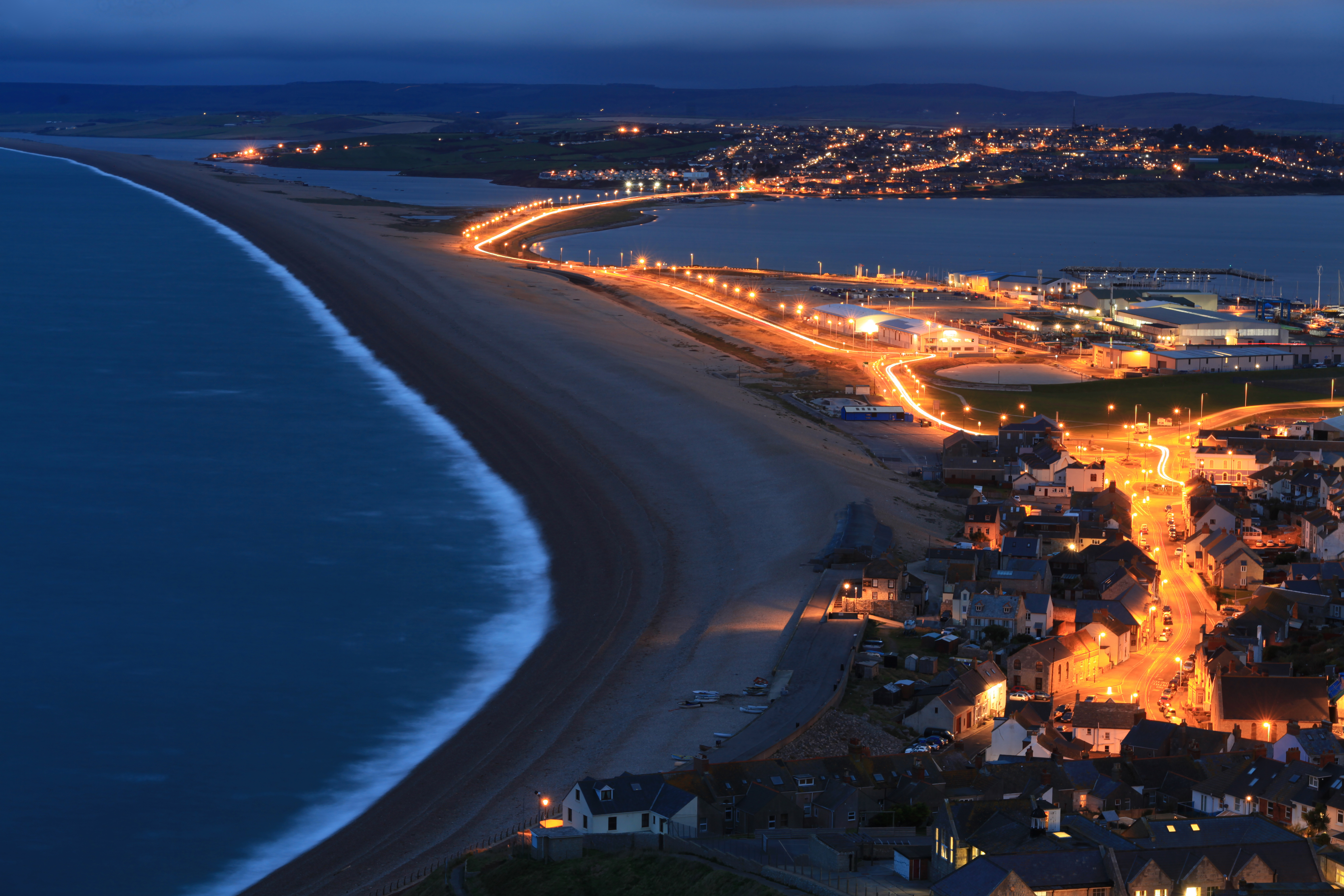
Where does our electricity come from? One answer might be the power stations, wind turbines and solar panels that generate it. You might even go as far as to say the wind, sun, water, biomass and gas powering those stations. Or even the network companies transporting that power around the country. But there’s also a very important middle-man in this process: electricity suppliers.
Most of Great Britain gets its power from one of the ‘Big Six’ energy suppliers, which buy electricity from the wholesale market and then sells it to consumers. However, with more businesses and consumers looking for less carbon-intense electricity sources, there are now a whole host of smaller companies taking on the incumbents and offering all-renewable electricity.
From Ovo to Bulb to Drax’s own Haven Power and Opus Energy, consumers and businesses have more and greener options than ever about where to buy their electricity, with many even offering 100% renewable electricity.
But how do these companies ensure the megawatts powering homes, offices and street lights come from renewable sources?
Cleaning up the river
The electricity we use doesn’t just flow through a single cable from a power station to our houses. It travels through what’s called the transmissions system, which is run by National Grid ESO and local distribution network operators.
Apart from off-grid installations like solar panels on buildings, some of which are unable to export their unused power, all the electricity generated by different sources around the country goes into this same system. It means megawatts generated by a wind turbine get mixed up with those generated by a nuclear reactor or a coal power station.
Think of it as a river. Although it is its own entity, it is fed by multiple streams of water coming from different sources. In the case of electricity, megawatts from various generators are fed into a central system, which then enter homes, offices and devices around the country.
So, what makes green power generated from renewable sources, green power used in homes?
Suppliers can’t control exactly what megawatts you use, but they can influence the makeup of the overall ‘river’ your electricity is pulled from by what electricity they agree to buy and offer to their customers.
Renewable suppliers match the amount of electricity their customers use with the amount they buy from renewable sources. So, if a home uses 4 megawatt-hours (MWh) a year, a supplier will need to ensure it buys an equal amount of power from National Grid, which National Grid sources from generators. If that supplier offers 100% renewable power, it will need to ensure it has the right kinds of deals in place with renewable generators to deliver that amount of power.
It means that while the river of electricity is still a mix from different streams, more of the water will come from renewable streams. Therefore, if more homes and businesses switch to renewable suppliers, more of the overall river will be renewable, which will in turn help to decarbonise the electricity system, enabling a lower-carbon economy.
But how does this fit into the existing electricity business and infrastructure?
But how do suppliers actually buy renewable power from generators?
The business of electricity
To understand how suppliers ensure they are buying renewable power you first need to understand how the business works. Or at least, how it used to. The most obvious place to start is with the generators.
Be they gas power stations or an offshore wind farm, the generator is where electricity is produced and are often owned by a supplier.
Suppliers can buy electricity from their own generators, often months or years ahead of delivery. But if there is a shortfall, the supplier can also buy electricity on the wholesale market, where other generators can sell their electricity.
Because suppliers are on a competitive market, their aim is to buy the electricity for the lowest possible price and sell it for more – but at a better rate than rivals. Measures like carbon prices or green incentives help lower the cost of renewable and low-carbon generation, and position it as a more economically viable purchase than more expensive fossil fuels like coal.
Renewable-only suppliers also want to buy electricity as cheap as possible and sell it as affordably as possible. But unlike standard suppliers they only buy electricity from renewable sources.
This can be done by purchasing electricity from independent renewable generators on the wholesale market, or arranged through what are known as Power Purchase Agreements (PPAs) – longer-term contracts between generators and suppliers agreeing on a specific amount of power.
The advantage of these for the renewable generator is it secures revenue for the future, while for the supplier it means a dependable source of electricity. For large installations, these deals are often signed before construction even begins to ensure investors there will be a return.
Transitioning to a low-carbon electricity system, however, is not just about suppliers buying more electricity from renewable sources.
The future of electricity suppliers
As more businesses, individuals and communities are becoming prosumers and generating their own electricity, the wider role of the supplier in the system is changing.
The government’s feed-in-tariffs financially reward customers for generating their own electricity, even if they don’t export it to the grid. But even small generators can sign deals with suppliers to sell electricity through schemes such as Good Energy’s SmartGen policy, which is open to generators with between 10 and 100 kilowatts (kW) of installed capacity. Similarly Opus Energy helps over 2,100 businesses sell more than 1,100 gigawatt-hours (GWh) of excess wind, solar, anaerobic digestion and hydro power.
For larger prosumer businesses, the relationship with suppliers and the grid is different. Rather than the traditional buying and selling of electricity, it requires a cooperative approach to understand how the prosumer can best utilise their assets.
Beyond increasing the amount of low-carbon electricity, decarbonising the electricity system also means making more efficient use of energy and managing the data that can help improve efficiency. Haven Power’s partnership with Thames Water sees it analyse an average of 68 million half-hour smart meter readings every year, using the data to help the company improve its billing and forecasting. As the wider system becomes more intelligent, suppliers will be able to better forecast how much electricity its customers use and help them reduce their consumption.
The role required of suppliers in a changing system will create opportunities for more renewable and efficient use of electricity. And empower more consumers to get their electricity from low-carbon sources that can help to make the whole country’s electricity greener.
Want a different perspective on the same story? Watch TV’s Jonny Ball explain.